Despite impressive improvements in the overall safety of anesthetized patients in the past two decades, brain injury remains a major and growing problem. This is demonstrated by the on-going American Society of Anesthesiologists closed-claims analysis. Brain injury (including brain damage, stroke & awareness) represents the single largest fraction (17%) of malpractice claims.1 Consistent with this finding are the recent large multicenter US cardiac2 and international non-cardiac surgery3 studies. The US study found a 6% incidence of major brain injury following myocardial revascularization, whereas the international study, performed on patients at least 60 years of age, noted an alarming 26% incidence of marked cognitive decline postoperatively. Although such postoperative deterioration has often been discounted as a transient epi-phenomenon, Newman et al.4 recently showed that it was highly predictive of neurocognitive deterioration five years later. Newman and colleagues5 have also shown that the deterioration is closely associated with a diminished quality of life and is age-related. Their work emphasizes that from a patient safety orientation, post-surgical brain injury is a growing socioeconomic as well as a potential medico-legal problem in our graying society.
The international study was also noteworthy for what it didn’t find or say. Surprisingly, neither episodes of systemic hypotension nor hypoxia were predictive of brain injury. Furthermore, though emboli clearly are involved in at least some of the brain injury after cardiac surgery, there was no mention of their involvement in the patients not exposed to the stresses of cardiopulmonary bypass. Suggestions regarding the cause(s) and cure(s) of this disturbing safety issue were not forthcoming.
However, suggestions of an etiology and a means for prevention are available. Moody et al.6 elegantly described the anatomical basis of the brain’s selective regional vulnerability to perfusion and oxygenation deficiency. Their examples illustrate that regional or focal ischemic/hypoxic brain injury can readily occur despite values of systemic arterial oxygen saturation and mean perfusion pressure close to population norms. In aging patients, the clinical significance of these anatomic features is accentuated by the narrowing and diminished compliance of cerebral arteries.
These factors predict that, especially in older patients, abnormalities in cerebral oxygenation occur independently from systemic measures and that they are associated with poorer outcome. Our experience with the new technology of cerebral oximetry is consistent with these predictions. We found a high (18%) incidence of marked (>25% below awake baseline) cerebrovenous oxygen desaturation in revascularization patients.7 Episodes of cerebral oxygen desaturation were not correlated with either systemic arterial or mixed venous oxygen saturation. In addition, we noted that desaturation of this magnitude was associated with disorientation8 and subtle neurologic signs of frontal lobe injury.9 Cerebral desaturation, as well, correlated with prolonged postoperative ventilatory support and length of intensive care stay.10 Our observations hopefully encourage a closer inspection of this technology and its patient safety implications.
Technology
Transillumination of body parts with visible or near-infrared (NIR) light having wavelengths between 650-1100 nm has become an established part of medical diagnosis. In the absence of light-absorbing materials (chromophores), some photons will penetrate tissue to considerable depths before resurfacing. Using sensitive photodiode detectors, light can be measured at considerable distances from the point of injection. However, the long, torturous paths taken by the scattered photons make them exceedingly sensitive to the optical properties of tissue. Even small amounts of a chromophore will cause wavelength-dependent photon absorption which yields a characteristic spectral signature from the emerging light.
In 1977, these concepts led Jöbsis11 to measure the NIR absorption spectrum of light passing through an infant’s head from temple to temple. He was able to detect increased light transmission during hyperventilation. Unfortunately, the large size of the adult brain precludes transillumination. However, within a decade Ferrari et al.12 and others had successfully measured hemoglobin oxygen saturation in the adult cerebral cortex using NIR reflected back to the scalp in the vicinity of the light source.
With reflectance cerebral oximetry, NIR photons are injected into the skin over the forehead. After scattering by skin, scalp, skull, dura and brain, some fraction of the injected photons return to the skin surface. By measuring the quantity of returning photons as a function of wavelength, one can characterize the spectral absorption of the underlying tissue and make sequential determinations of the average regional hemoglobin oxygen saturation (trended rSO2 index).
Intuitively, the greater the separation of source and detector, the greater the average depth of penetration. Injected photons traveling close to the surface will dissipate before arrival at a distant detector. A large source-detector separation is therefore biased against “shallow” photons except in the tissues directly under the source and detector. On the other hand, absorption by tissue chromphores reduces the probability of detecting deeply penetrating photons. Therefore, most detected photons necessarily take an optimum middle course. Cui et al.13 demonstrated this principle experimentally, using small optical absorbers placed in an intralipid-filled tank which approximated intracranial tissue. They found that photon penetration depth was a third of the source-detector separation distance and noted the same “banana-shaped” sensitivity distributions (Fig. 1) found by others14 using both experiments and computer simulations.
From diffusion theory applied to a turbid biological tissue, it is estimated that at a 4 cm source-detector separation, extracranial oxygen contamination accounts for 45% of the optical signal. Therefore, to minimize the significant influence of extracerebral oxygen, spatially resolved cerebral oximeters use two differentially spaced detectors to record photons reflected from extracranial (shallow) and intracranial (deep) tissues. Both equally sample shallow tissue layers directly under detectors, but intracranially reflected photons pass preferentially to the more distant detector. Subtraction of the shallow from the deep signal thus results in the recording of light reflected predominantly from the cerebral cortex. Thus, with a 3 cm shallow and 4 cm deep source-detector separation, at least 85% of the resultant signal appears to originate from brain tissue.
These estimates from computer simulations have been experimentally verified by Hongo et al.15 during carotid endarterectomy. They used a detector array on the forehead and selective injections of an infrared absorbing dye (indocyanine green) into the external and internal carotid arteries. Transient signal decrease was then observed at various source-detector separations. Dye injected into the external carotid resulted in similar signal decline in both shallow and deep detectors. In contrast, internal carotid injections produced larger change in the deep detector. Additionally, they found that source-detector separations of 3 and 4 cm were optimal for spatially resolving the optical signals to emphasize the superficial layers of the cerebral cortex. The minimal extracranial contamination of rSO2 index observed by Hongo et al. has very recently been independently confirmed.16
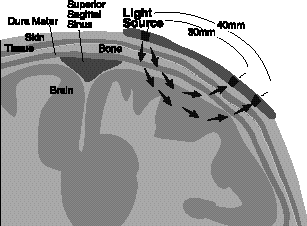
Figure 1. The characteristic banana-shaped mean photon paths for source-detector separations of 30 and 40 mm.
Technoclinical Controversy
The Somanetics INVOS 4100 Cerebral Oximeter is the cerebral oximeter currently cleared by the U.S. Food and Drug Administration for routine use in adult patients. It is designed specifically for measuring oxygen in the brain in the area underlying the sensor. It uses two wavelengths, 724 and 810 nm, to measure changes in regional hemoglobin oxygen saturation (rSO2 index). Like all other new technologies, the introduction of this device has spawned controversy from skeptical clinicians and competing technologies. Therefore, some of the controversial issues and limitations of the technology are presented here:
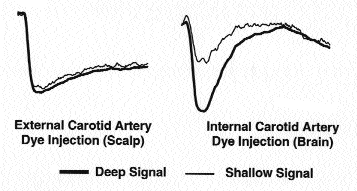
Figure 2. Since the rSO2 index is calculated from the shallow-deep difference, change was detected only with internal carotid injection of light absorbing dye.
1. Absolute vs. relative measurement
Because the Beer-Lambert Law governs the measurement of chromophore concentration using spectroscopy, knowledge of the photon path length is necessary for quantification of the absolute concentrations of oxyhemoglobin and reduced hemoglobin. However, if oxygen saturation is the final measurement and one assumes the path length of photons of each wavelength to be equal or proportional across subjects, then the path length factor may be removed from the equation. While these assumptions are generally true, the residual error would be quite high if only a single detector system were used. However, with a spatially-resolved cerebral oximeter, the shallow-deep detector difference represents optical signal change at two depths (i.e., the first derivative of optical density vs. depth of penetration). This method minimizes residual error and intersubject variation. A recent human study using a prototype NIR system capable of path length determination by phase-modulated light17, produced results similar to the spatially-resolved system currently available for clinical use.18
2. Scattering is wavelength-dependent
The algorithm used in the oximeter available for clinical use assumes that light scattering is not wavelength-dependent and that pathlengths for all wavelengths are equal. However, Benaron & Stevenson19 have shown that significant wavelength-dependent differences in scattering do occur in 3-10% of subjects. In the absence of direct pathlength measurements, the current clinical oximeter identifies this possibility through a signal quality index. A low index value indicates that the scattering coefficients for the two wavelengths used are unequal. Under these circumstances, the absolute value of hemoglobin oxygen saturation may be suspect, but the trending capability is unaffected.
3. rSO2 vs. SjVO2
Cerebral oximetry quantifies the oxyhemoglobin fraction within a small sample of cerebral cortex. In the absence of hemorrhage, the value represents the algebraic sum of intravascular arterial, capillary and venous oxyhemoglobin. Since the majority of the hemoglobin is found in the venous compartment, cerebral oximeter output is a venous-weighted average, distinct from both the pulse oximeter arterial and jugular bulb venous measures. Although rSO2 and jugular bulb saturation (SjVO2) often trend in parallel, there is no reason, a priori, to always expect close agreement. Thus, studies critical of rSO2 measurements, simply by virtue of an inconsistent association with SjVO2, 20,21 appear to be based on an inappropriate assumption. SjVO2 represents exclusively venous blood drained from a wide but uncertain territory. Jugular samples most often represent the venous admixture from the ipsilateral hemisphere, but significant variation in cerebral venous anatomy assures that this pattern is not a certainty. For example, Huang et al.22 describe the 12 most common patterns of venous drainage, none of which occurs in a majority of patients.23
In contrast, rSO2 is obtained from a small volume of frontal cortex. Marked differences between regional cortical and global hemispheric oxygenation may be expected, particularly in non-physiologic circumstances such as cardiopulmonary bypass. This measurement discrepancy has been demonstrated by Mutch et al.24 using jugular bulb oximetry and functional magnetic resonance imaging (fMRI) of oxy- and deoxyhemoglobin. During non-pulsatile perfusion, patchy regions of poorly oxygenated cortical tissue appeared in fMRI images that were invisible to the SjVO2 hemispheric average.
4. Normal rSO2 values may occur in brain-dead or dead subjects
Several reports25,26 have questioned the reliability of rSO2 measurements because values typically observed in conscious healthy subjects were also seen in brain dead or dead patients. As with rSO2-SjVO2 discrepancies, this apparent difficulty seems the result of an inappropriate expectation. Maeda et al.27 examined cerebral venous blood samples during 214 autopsies. Hemoglobin oxygen saturation ranged from 0.3 to 95%. Not surprisingly, the authors concluded that 1) oximetric profiles reflected, in large part, the specific circumstances of death as well as storage conditions thereafter, and 2) post-mortem oximetry was of limited value. It thus seems unreasonable to expect unperfused cerebral tissue to always have cerebral venous oxygen saturation within a predetermined narrow range. When thoughtfully applied as a trend monitor, the cerebral oximeter can detect declining cerebral perfusion resulting from progressive intracranial hypertension in both adults28 and children.29
5. The ratio of cerebral arterial to venous blood is not constant
The clinically available oximeter assumes a constant 25% arterial and 75% venous blood volume in its estimate of rSO2 via field saturation or fSO2 (fSO2=0.25SaO2+0.75SjVO2). A close correlation between the rSO2 index and fSO2 calculated from direct arterial and jugular venous measurements has been demonstrated in volunteers.30 However, the influence of shifts in this ratio have not been established. Such shifts may occur in extreme situations such as selective retrograde cerebral perfusion. In these cases, interpretation of the absolute rSO2 index is uncertain, but trended values still permit detection and correction of inadequate perfusion.31
6. Dual wavelength oximeters can’t measure abnormal hemoglobins or cytochromes
Carboxy- and methemoglobin confound both pulse oximeters32 and dual wavelength cerebral oximeters and may result in either under or overestimation of functional hemoglobin saturation. This limitation must be kept in mind during the use of these monitors in critical clinical settings.
Change in NIR absorption due to the redox state of the cytochrome aa3 copper moiety permits measurement of this respiratory chain terminal O2 acceptor. Such measurement currently requires sophisticated and expensive research spectrometers using four or more wavelengths. Although changes in the cerebral cytochrome signal distinct from those of hemoglobin oxygen saturation occur during hypoxia, validation, interpretation and clinical utility of this novel measure remain to be established.33
Clinical Application
Change in rSO2 is influenced both by oxygen delivery and consumption. Imbalance, or dysoxygenation, is manifested by rSO2 change. Both decreases and increases from baseline may signify dysfunction and developing pathology.34 Baseline values vary, in part, due to substantial heterogeneity in cerebral oxygen extraction efficiency. For example, we noted an awake preoperative rSO2 baseline of 65±9% (mean ± s.d.) in a cohort of 250 coronary artery surgery patients, yielding a wide “normal” (i.e. 2 s.d.) range of 47-83%. Furthermore, the relationship between a low absolute rSO2 and adverse neurologic outcome is complex. It depends on many variables such as the duration of the dysoxygenation, the brain temperature35, tissue optical density/photon path length36 and presence of potentially neuroprotective drugs.37 Yet, despite these theoretical limitations, abnormally low absolute rSO2 values have been associated with evidence of cerebral dysfunction38 and higher hospital costs.39 In monitoring applications, clinically significant dysoxygenation also has been defined in relative terms, i.e., as change from baseline. Studies on conscious individuals involved in +Gz acceleration,40 implantable cardioverter-defibrillator testing,41 tilt-table syncope testing42 and carotid endarterectomy under regional anesthesia43 all found signs of severely impaired cerebral function with a relative >25% rSO2 decline.
Emphasis on rate of change is particularly important in clinical situations that challenge rSO2 computational algorithm assumptions. For example, the marked increase in hemoglobin affinity for oxygen that occurs with hypocapnea, alkalosis or hypothermia may confound the interpretation of absolute rSO2 values. However, despite loss of baseline conditions, a sudden, precipitous rSO2 decline still indicates cerebral dysoxygenation in immediate need of correction. This view is supported by our extensive cerebral oximetric experience with transcranial Doppler-verified cerebral perfusion changes associated with deep hypothermic circulatory arrest in adults44 and children.45
Cerebral oximetric monitoring facilitates prompt correction of detected dysoxygenation. We have used a set of simple, cost-effective strategies to correct declining rSO2.46 These include increased inspired oxygen level, elevation of arterial carbon dioxide or arterial pressure, hemoglobin augmentation or anesthetic suppression of cerebral metabolism. Using these tools, we had 93% success in correcting dysoxygenation in a group of 630 myocardial revascularizations.
Conclusions
This brief review of cerebral oximetry has discussed the following concepts:
- Perioperative brain injury is a large and growing problem.
- Although its etiology is multifaceted, selective regional cerebral hypoxia and hypoperfusion are undoubtedly involved.
- Inadequate cerebral oxygenation or perfusion often can not be detected without directly monitoring some aspect of brain function.
- The cerebral oximeter currently available for clinical use can detect brain tissue at risk and assess intervention effectiveness in a timely fashion.
Although the efficacy of cerebral oximetry has been investigated most extensively during cardiothoracic surgery, recent studies have illustrated its potential in a wide range of clinical situations. Like most new technologies, early experience with cerebral oximetry has resulted in a series of anecdotal and retrospective efficacy studies such as those referenced here; prospective randomized cost-effectiveness or cost-benefit studies are lacking. However, the recently popularized evidence-based medicine approach essentially represents an unfunded mandate to manufacturers. Solutions to this expensive conundrum, perhaps involving non-traditional study designs and funding sources, will require close collaboration among manufacturers, health care professionals, administrators, and regulators. At this time, the preponderance of available evidence suggests that cerebral oximetry can improve patient safety through maintenance of cerebral oxygen balance in critical clinical situations.
Dr. Edmonds is Professor and Director of Research in the Department of Anesthesiology, University of Louisville School of Medicine. A neuropharmacologist/ physiologist interested in intraoperative neuromonitoring and neuroprotective strategies, he is a founding fellow of the American Society of Neurophysiological Monitoring. Disclosure: The author is a paid consultant to several neuromonitoring companies including Aspect Medical, Datex-Ohmeda, Nicolet Biomedical and Somanetics. Also, Baxter Pharmaceuticals and Electrocap International have provided research support. However, neither the author nor any family member has any other financial relationship with any of these companies.
References
- Caplan RA. The ASA closed claims project: lessons learned. ASA Annual Refresher Course, 1998:221.
- Roach GW, Kanchuger M, Mora-Mangano C, Newman M, Nussmeier N, Wolman R, Aggarwal A, Marschall K, Graham SH, Ley C. Adverse cerebral outcomes after coronary bypass surgery. New Engl J Med 1996;335:1857-63.
- Moller JT, P Cluitmans, Rasmussen LS, Houx P, Rasmussen H, Canet J, Rabbit P, Jolles J, Larsen K, Hanning CD, Langeron O, Johnson T, Lauven PM, Kristensen PA, Biedler A, van Beem H, Fraidakis O, Silverstein JH, Beneken JEW, Gravenstein JS. Long-term postoperative cognitive dysfunction in the elderly: ISPOCD1 study. Lancet 1998;351:857-61.
- Newman MF, Kirchner J, Phillips-Bute B, Phillips E, Han D, Braudet B, Grigore AM, Reves JG, Blumenthal JA. Perioperative neurocognitive decline predicts long-term (5-year) neurocognitive deterioration after CABG. Anesth Analg 1999;88:SCA93.
- Newman MFk Croughwell ND, Blumenthal JA, White WD, Lewis JB, Smith LR, Frasco PF, Towner EA, Schell RM, Hurwitz BJ, Reves JG. The effect of aging on cerebral autoregulation during cardiopulmonary bypass: association with postoperative cognitive dysfunction. Circulation 1994;90:II243-9.
- Moody DM, Bell MA, Challa VR. Features of the cerebral vascular pattern that predict vulnerability to perfusion or oxygenation deficiency. Amer J Neuroradiol 1990;11:431-9.
- Edmonds HL Jr, Toney KA, Thomas MH, Pollock SB Jr, Strickland TJ. Neuromonitoring cost-benefit for adult cardiac surgery. Anesthesiol 1997;87(3A):A426.
- Edmonds HL Jr, Sehic A, Pollock SB Jr, Ganzel BL. Low cerebrovenous oxygen saturation predicts disorientation. Anesthesiol 1998;89(3A):A941.
- Edmonds HL Jr, Thomas MH, Sehic A, Pollock SD, Ganzel BL. Cerebral oxygen desaturation during myocardial revascularization is associated with frontal lobe injury. Anesth Analg 1998;86:SCA13.
- Edmonds HL Jr, Yu Q-J, Ganzel BL. Cost of cerebral macroembolization and oxygen desaturation during myocardial revascularization. Stroke 1998;29:2238.
- Jöbsis FE. Noninvasive, infrared monitoring of cerebral and myocardial oxygen sufficiency and circulatory parameters. Science 198:1264-l267, l977.
- Ferrari M, Zanette E, Giannini I. Effects of carotid artery compression test on regional cerebral blood volume, hemoglobin oxygen saturation, and cytochrome c oxidase redox level in cerebrovascular patients. Adv Exp Med Biol 1986;200:213-220.
- Cui W, Kumar C, Chance B. Experimental study of migration depths for the photons measured at sample surface. SPIE 1991;1431:180-191.
- McCormick PW, Stewart M, Goetting MG, Dujovny M, Lewis G, Ausman JI. Noninvasive cerebral optical spectroscopy for monitoring cerebral oxygen delivery and hemodynamics. Crit Care Med 1991;19:89-97.
- Hongo K, Kobayashi S, Okudera H, Hokama M, Nakagawa F: Noninvasive cerebral optical spectroscopy for monitoring cerebral hemodynamics – basic study using indocyanine green. Neurol Res 17:89-93, 1995.
- Samra SK, Stanley JC, Zelenock GB, Dorje P. An assessment of contributions made by extracranial tissue during cerebral oximetry. J Neurosurg Anesth 1999; 11:1-5.
- Levy WJ, Carpenter J, Fairman RM, Golden MA, Zager E: The calibration and validation of a phase-modulated near-infrared cerebral oximeter. Anesthesiology 1998;89(3A):A539.
- Henson LC, Cartwright C, Chlebowski, SM,Kolano JW, Ward DS. Estimation of jugular venous O2 saturation from cerebral oximetry or arterial O2 saturation during isocapnic hypoxia. Anesthesiology 1997;87(3A):A402.
- Benaron DA, Stevenson DK. Resolution of near-infrared time-of-flight brain oxygenation imaging. Adv Exp Med Biol 1994;345:609-17.
- Grubhofer G, Lassnigg A, Manlik F, Marx E, Trubel W, Hiesmayr M. The contribution of extracranial blood oxygenation of near-infrared spectroscopy during carotid thrombendarterectomy. Anaesthesia 1997;52:116-120.
- Lewis SB, Myburgh JA, Thornton EL, Reilly PL. Cerebral oxygenation monitoring by near-infrared spectroscopy is not clinically useful in patients with severe closed-head injury: a comparison with jugular venous bulb oximetry. Crit Care Med 1996;24:1334-38.
- Huang YP, Okudera T, Ohta T, Robbins A. Anatomic variations of the dural venous sinuses. In The Cerebral Venous System and Its Disorders. (Eds. JP Kapp, HH Schmidek) Orlando: Grune & Stratton.1984, pp 109-167.
- Durgun B, Ilgit ET, Cizmeli MO, Atasever A. Evaluation by angiography of the lateral dominance of the drainage of the dural venous sinuses. Surg Radiol Anat 1993;15:125-30.
- Mutch WAC, Ryner LN, Kozlowski P, Scarth G, Warrian RK, Lefevre GR, Wong TG, Thiessen DB, Girling LG, Doiron L, McCudden C, Saunders JK. Cerebral hypoxia during cardiopulmonary bypass: a magnetic resonance imaging study. Ann Thorac Surg 1997;64:695-701.
- Muellner T, Schramm W, Kwasny O, Vecsei V. Patients with increased intracranial pressure cannot be monitored using near-infrared spectroscopy. Brit J Neurosurg 1998;12:136-9.
- Schwarz G, Litscher G, Kleinert R, Jobstmann R. Cerebral oximetry in dead subjects. J Neurosurg Anesth 1996;8:189-193.
- Maeda H,Fukita K, Oritani S, Ishida K, Zhu B-L. Evaluation of post-mortem oxymetry with reference to causes of death. Forensic Sci Internat 1997;87:201-210.
- Holzscuh M, Brawanski A, Metz C, Woertgen C. Long-term comparison of tissue oxygen pressure and cerebral oxygen saturation in head injury. J Neurotrauma 1995;12:465.
- Nemoto EM, Adelson Dl. Painter M. Cerebral oximetry in head-injured children. J Neurosurg Anesth 1998;10:283.
- Pollard V, Prough DS, DeMelo E, Deyo DJ, Uchida T, Stoddart HF. Validation in volunteers of a near-infrared spectroscope for monitoring brain oxygenation in vivo. Anesth Analg 1996;82:269-77.
- Blas ML, Lobato EB, Martin T. Noninvasive infrared spectroscopy as a monitor of retrograde cerebral perfusion during deep hypothermia. J Cardiothorac Vasc Anesth 1999;13:244-5.
- Sprung J, Bourke DL, Mackenzie CF, Thomas P. Chronic methemoglobinemia: improving hemoglobin saturation monitoring during anesthesia. J Clin Monit 1994;10:267-9.
- Matsumoto H, Oda T, Hossain M, Yoshimura N. Does the redox sate of cytochrome aa3 reflect brain energy level during hypoxia? Anesth Analg 1996;83:513-8.
- McCormick PW, Stewart M, Goetting MG, Balakrishnan G. Regional cerebrovascular oxygen saturation measured by optical spectroscopy in humans. Stroke 1991;22:596-602.
- Kurth CD, Levy W, Chance B. Oxygen metabolism during circulatory arrest. J Neurosurg 1994;81:330.
- Kurth CD, Steven JM, Swedlow D. New frontiers in oximetry. Am J Anesthesiol 1996;23:169-175.
- Hoffman WE, Charbel FT, Edelman G, Misra M, Ausman JI. Comparison of the effect of etomidate and desflurane on brain tissue gases and pH during prolonged middle cerebral artery occlusion. Anesthesiol 1998;88:1188-94.
- BhaskerRao B, VanHimbergen D, Jaber S, Ali AT, Edmonds HL Jr, Pagni S, Koenig S, Spence PA. Evidence for improved cerebral function after minimally invasive bypass surgery. J Cardiac Surg 1998;13:27-31.
- Edmonds HL Jr, Austin EH III, Seremet V, Niznik G, Sehic A, Audenaert SM, Sowell MK. Cost-benefit analysis of neuromonitoring for pediatric cardiac surgery. Anesth Analg 1997;84:SCA22.
- Chelette TL, Albery WB, Esken RL, Tripp LD. Female exposure to high G: performance of simulated flight after 24 hours of sleep deprivation. Aviation, Space & Environ Med 1998;69(9):862-8.
- Singer I, Edmonds HL Jr. Changes in cerebral perfusion during third-generation implantable cardioverter-defibrillator testing. Am Heart J 1994;127:1052-7.
- Singer I, Edmonds HL Jr. Head-up tilt testing predicts syncope during ventricular tachycardia in implantable cardioverter-defibrillator patients. J Interven Cardiol 1998; 11(3):205-11.
- Roberts KW, Crnkowic AP, Linneman LJ. Near-infrared spectroscopy detects critical cerebral hypoxia during carotid endarterectomy in awake patients. Anesthesiol 1998; 89(3A):A934.
- Ganzel BL, Edmonds HL Jr, Pank JR, Goldsmith LJ. Neurophysiological monitoring to assure delivery of retrograde cerebral perfusion. J Thorac Cardiovasc Surg 1997;113:748-57.
- Austin EH III, Edmonds HL Jr, Seremet V, Niznik G, Sehic A, Audenaert SM, Sowell MK. Benefit of neuromonitoring for pediatric cardiac surgery. J Thorac Cardiovasc Surg 1997;114:707-17.
- Edmonds HL Jr, Rodriguez RA, Audenaert SM, Austin EH, Pollock SB Jr, Ganzel BL. The role of neuromonitoring in cardiovascular surgery. J Cardiothorac Vasc Anesth 1996;10(2):15-23.